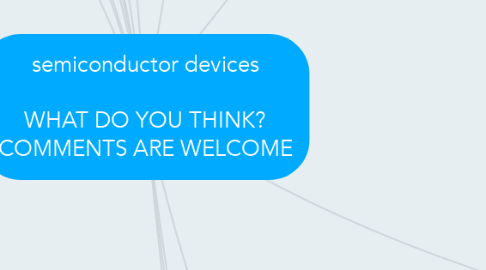
1. III-V
1.1. properties
1.2. applications
2. silicon
2.1. applications
2.1.1. mems/nems
2.1.1.1. mechanical stress testing
2.1.1.2. pressure sensor
2.1.1.3. biomimetic
2.1.1.3.1. mems sensor flow-related controll
2.1.1.4. acceleration sensor: pull-in instability of paddle-type nems sensor
2.1.2. electronics
2.1.2.1. transistor
2.1.2.1.1. logic transistor
2.1.3. self-assembly milli to nano scale
2.2. properties
2.2.1. mechanical
2.2.1.1. gauge factor: 200
2.2.1.2. young's modulus: 130-188 GPa
3. MoS2
3.1. properties
3.1.1. piezoelectricity: piezoelectric coefficient: e11 = 2.9 × 10–10 C m−1
3.1.1.1. piezoelectricity of singl layer mos2 for energy conversion and piezotronics
3.1.2. electrical
3.1.2.1. screening
3.1.2.2. bandgap: intrinsic direct 1.8 eV indirect bandgap 1.2 eV
3.1.2.2.1. bilayer
3.1.2.2.2. strain induced bandgap tuning
3.1.2.3. mobility @ RT: 200 cm^2/Vs
3.1.2.4. on/off ration: 1x10^8
3.1.2.5. doping
3.1.2.6. electrical properties on hBN
3.1.2.7. effect of interlayer coupling on electrical properties
3.1.3. mechanical
3.1.3.1. thickness: 0.65nm
3.1.3.2. mechanical
3.1.3.3. pressure confinement effect
3.1.3.4. strain effect on effective mass
3.1.3.5. gauge factor
3.1.3.5.1. bi-layer: 230
3.1.3.5.2. bulk: 200
3.1.3.6. uniaxial and biaxial strain
3.1.3.7. wettability
3.1.3.8. friction
3.1.4. optical
3.1.4.1. electroluminescence
3.1.4.2. raman spectroscopy
3.1.4.2.1. MoS2 488nm laser
3.1.5. heat conductance: 7.45 W/mK @ 300K suspended monolayer: 34 W/mK suspended multilayer: 52 W/mK
3.1.6. chemical
3.1.6.1. one layer of Mo -sandwiched between two layers of S by covlent bonding packed in hexagonal arrangement
3.1.6.2. sheets held together by weak van der Waals interaction
3.1.6.3. lattice constant: 3.160 Å
3.1.7. thermal
3.2. application
3.2.1. chemical vapor sensor (comparison with graphene devices)
3.2.2. transistor
3.2.2.1. channel
3.2.2.2. contacts
3.2.2.2.1. injet printed Ag contacts
3.2.2.3. CVD grown
3.2.2.3.1. multilayer
3.2.2.3.2. single layer
3.2.2.4. flakes
3.2.2.4.1. single layer
3.2.2.5. flexible transistor
3.2.2.5.1. on polyimide
3.2.2.6. charge trapping at the interface
3.2.2.7. thin film transistor
3.2.2.8. strain sensing
3.2.2.9. self-screened transistor with bottom graphene electrode
3.2.2.10. transfer characteristics
3.2.2.11. suspended transistor
3.2.2.12. intrinsic origin of the hysteresis suspended mos2
3.2.3. supercondictivity
3.2.4. MEMS/NEMS
3.2.4.1. membrane
3.2.4.1.1. resonator
3.2.4.1.2. nanopores open atom by atom
3.2.4.1.3. direct and scalable CVD membranes
3.2.4.1.4. hydrogen separation
3.2.4.1.5. water desalination
3.2.4.2. flexible MoS field-effect transistor for gate-tunable strain sensor
3.2.4.3. bio/medicin
3.2.4.3.1. review: bio sensors
3.2.4.3.2. functionalication rection between mos2 and thiole
3.2.4.4. sensors
3.2.4.4.1. synthesis and sensor applications of mos2-based nanocomposites
3.2.4.4.2. gas sensor
3.2.4.4.3. tactile sensor for electronic skin
3.2.4.5. bending response theory
3.2.5. sensing
3.2.6. supercapacitor
3.2.6.1. MoS2-Graphene composite coin cell supercapasitor
3.2.7. heterostructure
3.2.7.1. electric-field and strain-tunable mos2/h-bn/graphene vertical heterostructure
3.2.7.2. review
3.2.7.3. one-dimensional electrical contacts to mos2 heterostructure
3.3. contacting
3.3.1. graphene electrode
3.3.2. contact resistance
3.3.3. carrier transport at the metal-mos2 interface
3.3.4. metal contacts
3.3.5. vam der waals interaction and lattice mismetch at mos2/metal interfaces
3.3.6. chromium as ideal contact metal
3.4. transfer
3.4.1. wet etch method
3.4.1.1. BOE etch
3.4.1.1.1. spin-coat: ar-p 649.04 30s@1800rpm,2 etch SiO2 with BOE
3.4.1.2. NaOH etch
3.4.1.2.1. spin-coat: ar-p 649.04 30s@1800rpm,2 etch SiO2 with NaOH
3.4.1.3. KOH etch
3.4.1.3.1. spin-coat: ar-p 649.04 30s@1800rpm,2 cratch the corners of the chip place a drop of KOH etch sio2
3.4.2. ultrasound method
3.4.3. pdms stamp
3.4.3.1. mos2 flake
3.4.3.2. polymer free large-area transfer for transistors fragmented mos2
3.4.4. fast Seak and peal in water drop with Polysterene in Touluene polymer
3.4.5. exfoliation
3.4.5.1. liquid phase exfoliation
4. hexagonal boron nitride (hBN)
4.1. porperties
4.1.1. impermeability to everything except to protons
5. carbon nanotubes
5.1. properties
5.2. applications
5.2.1. mems/nems
5.2.1.1. switch
5.2.1.2. pressure sensor
5.2.1.2.1. pmma based
5.2.2. electronics
5.2.2.1. transistor
5.2.2.1.1. logic transistor
5.3. synthesis
6. graphene
6.1. transfer
6.1.1. wet-etch method
6.1.1.1. dry transfer with spacer substrate
6.1.1.2. copper on sio2 pattern graphene underetch copper leave graphene on sio2
6.1.2. cleaning
6.1.2.1. dry-cleaning with active graphite
6.1.2.2. polymer scaffolds
6.1.2.3. thermal annealing at 300°C for 3 hours under UHV
6.1.2.4. metal cleaning, crackless, wrinkleless
6.1.2.5. acetic acid and methanol
6.1.2.6. residue reduction
6.1.3. exfoliation
6.1.3.1. on SiC
6.1.3.2. liquid phase exfoliation
6.1.3.3. polymer nanoparticles assisted exfoliation
6.1.4. nano imprint
6.1.5. glue on substrate
6.1.5.1. epoxy
6.1.6. copper evaporation
6.1.7. carbon atom diffusion through copper
6.1.8. large area patterning transfer with holographic lithography
6.1.9. polymer assisted
6.1.9.1. polymer free transfer with cellulose acetate
6.1.9.2. dry PI polymer transfer (copper reuse)
6.1.9.3. roll-to-roll transfer method
6.1.9.4. pet assisted transfer method
6.1.9.5. soak and peel method
6.1.9.6. dry pdms stamp transfer
6.1.9.6.1. pdms stamp with o2 plasma before stamp on cu foil: 30W, 15s O2 plasma enhance adhesion
6.1.9.6.2. pdms transfer without pmma pdms remove by methylene chloride
6.1.9.6.3. large area suspended graphene transfer with pdms
6.1.9.6.4. low temperature, metal assisted
6.1.9.7. dry thermal release tape
6.1.9.8. vacuum assisted transfer -remove of adsorbants - use standard wet transfer
6.1.9.9. pmma and ab-glue
6.1.9.10. spin-coater assisted transfer to polymer substrate
6.1.9.11. resiude fre pmma remove with ar+ ion beam
6.1.10. by oxidation-assited water intercalation
6.1.11. etch free-transfer
6.1.11.1. transfer free suspended graphene
6.1.11.2. electrochemical transfer method
6.1.11.2.1. bubble method
6.1.11.2.2. agarose gel method
6.1.11.2.3. pdms assisted without pores
6.1.11.2.4. electro-exfoliating grapene from graphite
6.1.11.2.5. bubble transfer with polymer support with inclosed air bubble
6.1.11.3. polyvinyl alcohol (PVA) film
6.1.11.4. dry-transfer
6.1.11.4.1. dry transfer with bN
6.1.11.4.2. selective dry transfer
6.1.11.4.3. dry electrostatic method
6.1.11.5. water-mediated and instataneous transfer
6.1.12. support free transfer with SAM modified substrate
6.1.13. wetting assisted transfer
6.1.14. polymer free transfer
6.1.14.1. graphene growth on patterned mo and removal of ma with sulfuric acid
6.1.14.2. with Ti as transfer layer removed with hf
6.1.14.3. using hexane
6.2. properties
6.2.1. mechanical
6.2.1.1. intrinsic strength: prestine graphene 90 - 121 GPa (30 N/m)
6.2.1.1.1. defective graphene ~50 GPa (18 N/m)
6.2.1.1.2. policrystalline
6.2.1.2. young's modulus: prestine and cvd graphene 1 TPa
6.2.1.3. Stretchability: 20%
6.2.1.4. impermeability to everything but protons
6.2.1.5. strain
6.2.1.5.1. uniaxual strain - deformation
6.2.1.5.2. uniaxial strain in bilayer graphene unisotropic phonon softening
6.2.1.6. Thickness: 0.34 nm
6.2.1.7. defect introduction through Argon irradiation
6.2.1.8. crack propagation
6.2.1.8.1. self healing of cracks
6.2.1.8.2. fracture of graphene (review)
6.2.1.9. wet adhesion
6.2.1.10. adhesion
6.2.1.10.1. strong adhesion to sio2
6.2.1.10.2. weak adhesion on pdms --> low surface energy
6.2.1.10.3. temperature-dependent adhesion on a trench
6.2.1.11. suspended
6.2.1.11.1. <10nm thick graphene: spring constant: 1-5 N/m
6.2.1.12. critical temp and radus for buckling
6.2.1.13. topography
6.2.1.14. small scale pull-in instability and vibration
6.2.1.15. gauge factor
6.2.1.15.1. exfoliated graphene
6.2.1.15.2. cvd graphene
6.2.2. electrical
6.2.2.1. high electron mobility
6.2.2.1.1. on bulk
6.2.2.1.2. suspended prestine at room temperature: 230000 cm2/Vs
6.2.2.1.3. suspended low temperature: order of 1000000 cm2/Vs
6.2.2.1.4. mobility extraction
6.2.2.1.5. CVD graphene on hBN: 350000 cm^2/Vs
6.2.2.1.6. CVD-graphene
6.2.2.1.7. exfoliated
6.2.2.2. screening
6.2.2.2.1. on hBN
6.2.2.3. electrical field
6.2.2.4. electrostatic
6.2.2.5. sheet resistance: 500 Ohm/square (wet transfer)
6.2.2.5.1. multilayer
6.2.2.5.2. depending on transfer
6.2.2.6. band structure
6.2.2.6.1. bandgap
6.2.2.7. breakdown current density
6.2.2.7.1. nanoribbons: width 16nm: 10^8 A/cm^2
6.2.2.8. effect of humidity on electrical properties
6.2.2.9. effect of interlayer coupling on electrical properties
6.2.2.10. surface electrical properties
6.2.3. optical
6.2.3.1. transparency: mono layer 97.7%
6.2.3.1.1. multilayer
6.2.3.1.2. fine structure constant
6.2.3.2. reflection: few layer: <0.1% > 10 layer: 2%
6.2.3.3. absorption: 300 - 2500 nm peak at 270 nm
6.2.3.4. photoresponse
6.2.3.5. raman
6.2.3.5.1. graphene 514nm and 633 nm laser
6.2.3.6. absorption: 2.3%
6.2.4. piezoresistivity
6.2.4.1. positive piezoconductive
6.2.5. chemical
6.2.5.1. hexagonal honeycomb lattice of carbon atoms in sp2 hybridization
6.2.5.2. remaining pz forms C-C pi bond
6.2.5.3. two atom A and B unit cell
6.2.5.4. superhydropobic graphene
6.2.5.4.1. superhydrophobic to superhydrophilic
6.2.5.5. intersurface interaction
6.2.6. thermal
6.2.6.1. exfoliated graphene
6.2.6.1.1. suspended heat conductivity: 5150 W/mK
6.2.6.1.2. thermal conductvity of suspended graphene with defect graphene
6.2.6.1.3. heat transport
6.2.6.1.4. thermal expansion coefficient: -7 × 10−6 K−1
6.2.6.2. CVD-graphene
6.2.6.2.1. suspended heat conductivity: 5150 W/mK
6.3. applications
6.3.1. mems/nems
6.3.1.1. membrane
6.3.1.1.1. pressure sensor
6.3.1.1.2. switch
6.3.1.1.3. accelerometer
6.3.1.1.4. loudspeaker
6.3.1.1.5. resonator
6.3.1.1.6. memory device
6.3.1.1.7. detection
6.3.1.1.8. with local gate control
6.3.1.1.9. electricity generation
6.3.1.1.10. TMD growth on suspended graphene
6.3.1.1.11. suspended graphene
6.3.1.1.12. membrane fabrication with tunable structures
6.3.1.1.13. strain sensor
6.3.1.1.14. microphone
6.3.1.1.15. fabrication
6.3.1.1.16. bio and medicin
6.3.1.1.17. characterization
6.3.1.1.18. properties
6.3.1.1.19. water desalination using nanoporous graphene
6.3.1.2. piezoelectric strain gauge
6.3.1.3. force sensor
6.3.1.4. nano bots
6.3.1.5. mechanical control of graphene on pyramidal structures
6.3.1.6. mos2 chemical vapor sensor (comparison with graphene devices)
6.3.1.7. hall sensor
6.3.1.7.1. quantum hall effect
6.3.1.8. transparent micro heater
6.3.1.9. tactile sensing with array of graphene woven nanofabrics
6.3.1.10. chemical sensor
6.3.1.10.1. gas sensor
6.3.1.11. control of nitrogen-vacancy defect emission
6.3.1.12. bio
6.3.1.12.1. bioanalytical applications
6.3.1.12.2. biosensor
6.3.1.12.3. bio-inspired strain sensors
6.3.1.12.4. biomedical applications
6.3.1.13. pressure sensor
6.3.1.13.1. pressure sensor with si3n4 membare and graphene strain elements
6.3.1.13.2. biomedical pressure sensor
6.3.1.13.3. electronical skin high speed rapid response criss cross graphene pattern
6.3.1.14. strain sensor for human motion monitoring
6.3.1.15. micromechanics
6.3.1.15.1. measurement of nanocrystalline graphnee
6.3.2. electronics
6.3.2.1. transistor
6.3.2.1.1. bilayer transistor
6.3.2.1.2. nanoribbon transistor
6.3.2.1.3. rf transistor
6.3.2.1.4. gbt (tunneling transistor)
6.3.2.1.5. Graphene field-effect device (GFET)
6.3.2.1.6. characterisation
6.3.2.1.7. terahetz detector
6.3.2.1.8. flexible
6.3.2.1.9. large scale integration
6.3.2.1.10. suspended
6.3.2.2. diode
6.3.2.3. circuits
6.3.2.3.1. flexible
6.3.2.4. solar cells
6.3.2.4.1. graphene molecules
6.3.2.5. supercapacitor
6.3.2.5.1. flexible
6.3.2.5.2. 3d graphene-based for application in supercapacitors
6.3.2.6. flexible electronics
6.3.2.6.1. tunable filed effect properties - low k-dielectric
6.3.3. folding
6.3.3.1. liquid evaporation driven
6.3.4. composite materials
6.3.4.1. graphene polymer Transparent, Flexible, and Conducting Films
6.3.5. optics
6.3.5.1. optoelectonics
6.3.5.1.1. photodetector
6.3.5.1.2. selectively enhanced photocurrent on twisted bilayer
6.3.5.2. photonics
6.3.5.3. light emission from graphene
6.3.5.3.1. nanocrystalline graphene
6.3.5.4. macroscopic and direct light propulsion of bulk graphene
6.3.6. heterostructures
6.3.6.1. graphene/hBN
6.3.6.2. electric-field and strain-tunable mos2/h-bn/graphene vertical heterostructure
6.3.6.3. graphene based heterostructure
6.3.7. superhydrphobic graphene
6.3.8. high temperature thin film devices
6.3.9. filtration and desalination of water
6.3.10. sensing
6.3.11. contacts
6.3.11.1. flexible
6.3.12. stability of few layer graphene doped with gold chloride
6.4. contacting
6.4.1. as electrode for mos2
6.4.2. interconnects
6.4.3. contact resistance
6.4.4. bottom graphene electrode
6.5. review
6.6. passivation
6.7. mulitlayer graphene
6.7.1. layer-by-layer stacking
6.7.1.1. stacking on copper without removing top pmma
6.7.1.2. stacking and removing pmma on substrate layer-by-layer
6.7.1.3. problem: increasing roughness
6.7.2. bernal stacked
6.8. graphene oxid (GO)
6.8.1. properties
6.8.1.1. mechanical
6.8.1.1.1. young's modulus: 0.15 +- 0.03 TPa
6.8.1.1.2. intrinsic strength: 4.4 +- 0.6 GPa (3.1 +- 0.4 N/m)
6.8.1.1.3. thickness: 0.7 nm
6.8.2. application
6.8.2.1. membrane
6.8.2.1.1. oil and water separation
6.9. graphene ink
6.9.1. properties
6.9.1.1. improve by laser-annealing
6.10. analytics
7. OSTE+
7.1. application
7.1.1. robust microdevices
7.1.2. improved photo structuring
8. WSe2
8.1. applications
8.1.1. light emitting tunneling transistor
9. WS2
9.1. properties
9.1.1. optical
9.1.1.1. photolminescence
9.2. contacting
9.2.1. chromium as ideal contact metal
9.3. applications
9.3.1. photodetector
9.3.2. mems/nems
9.3.2.1. gas sensor
9.3.2.2. humidity sensor
10. fabrication processes
10.1. Evaporation
10.2. Sputtering
10.3. Wet etching
10.3.1. copper
10.3.1.1. Sodiumpersulfate 20g/500ml H2O
10.3.1.2. FeCl3 8%: 20g FeCl3 + 230g H2O
10.3.2. sio2
10.3.2.1. Buffered oxide etch (BOE) 5:1 Buffered oxide etch (BOE), 5:1 Buffered HF, (5 40% NH4F:1 49%HF): 100 nm/min (thermal oxide)
10.3.2.2. concentrated HF (49%)
10.3.2.3. 10:1 HF 10 H2O: 1 HF: 23nm/min (thermal oxide)
10.3.2.4. HF vapor HF + H2O vapor, 1cm over dish with 49% HF: 66 nm/min (thermal oxide)
10.3.3. silicon nitride (Si3N4)
10.3.3.1. Buffered oxide etch (BOE), Buffered HF 5:1 Buffered oxide etch (BOE), 5:1 Buffered HF, (5 40% NH4F:1 49%HF): 60 nm/min (PECVD)
10.3.3.2. phosphoric acid
10.3.4. Si
10.3.4.1. KOH Isotropic, 30% by weight, 80°C: 1100 nm/min
10.3.5. poly si
10.3.5.1. KOH Isotropic, 30% by weight, 80°C: >1000 nm/min
10.3.6. Aluminum
10.3.6.1. KOH 30% by weight, 80°C: >800 nm/min
10.4. Dry etching
10.4.1. graphene
10.4.1.1. RIE: O2 80sccm O2 57mtorr 80W 20s
10.4.2. sio2
10.4.2.1. RIE: CF4 +O2 60mtorr 100W 44 nm/min (thermal oxide)
10.4.2.2. RIE: SF6 + Ar 40sccm SF6 30sccm Ar 10mtorr 50W 33nm/min
10.4.3. si
10.4.3.1. RIE: SF6 + Ar 40sccm SF6 30sccm Ar 10mtorr 50W 1500nm/min
10.4.4. Si3N4
10.4.4.1. RIE: SF6 + Ar 40sccm SF6 30sccm Ar 10mtorr 50W 150nm/min
10.4.5. mos2
10.4.5.1. RIE: BCl3 + Ar 15sccm BCl3 60sccm Ar 0.6 Pa 50W 5min
10.4.5.2. RIE: Ar coupled plasma RIE (CCP-RIE) 100 sccm Ar 10 Pa 50W > 90s
10.4.5.3. Layer by layer etching with cl and ar
10.5. Photolithography
10.5.1. photoresists
10.5.1.1. positive exposed to light becomes soluble unexposed remains insoluble
10.5.1.1.1. SPR 700-1.2
10.5.1.1.2. LOR 5A
10.5.1.2. negative exposed to light becomes insoluble unexposed is dissolved by developer
10.5.1.2.1. OSTE+
10.5.1.2.2. SU-8
10.5.2. systems
10.5.2.1. karl suss MJB-3 mask aligner
10.5.2.2. karl suss MA6/BA6 mask aligner
10.6. E-beam lithography
10.6.1. e-beam resists
10.6.1.1. negative exposed to e-beam becomes insoluble unexposed is dissolved by developer
10.6.1.1.1. AZ nLof 2007
10.6.1.1.2. su-8
10.6.1.2. positive exposed to e-beam becomes soluble unexposed remains insoluble
10.6.1.2.1. AR-P 679.02
10.6.1.2.2. AR-P 649.04
10.6.1.2.3. AR-P 617.14
10.6.2. systems
10.6.2.1. Raith
10.6.2.2. FEI + Raith Elphy Quantum
10.6.3. graphene fabrication
10.6.4. graphen small hole kitting
10.7. Plasma enhanced chemical vapor deposition (PECVD)
10.8. Critical point drying (CPD)
10.9. atomic layer deposition (ALD)
10.9.1. graphene
10.9.1.1. selective deposition of al2o3 on graphene
10.9.2. mos2 self-limiting
10.10. annealing
10.10.1. rapid thermal annealing (RTA)
10.10.2. forming gas annealing
10.10.2.1. system
10.10.2.1.1. hereus oven
10.10.3. remove PMMA (200nm): Haereus oven ramp: 10°C/min 450°C for 1h Ar/H2 (5%) (3 l/min / 0.1 l/min)
10.11. wafer dicing
10.12. spin-coating
10.13. synthesis
10.13.1. chemical vapor deposition (CVD)
10.13.1.1. Thermal chemical vapor deposition (thermal CVD)
10.13.1.1.1. graphene
10.13.1.1.2. mos2
10.13.1.1.3. TMDs
10.13.1.1.4. hBN
10.13.1.2. Plasma enhanced chemical vapor deposition (PECVD)
10.13.1.2.1. graphene
10.13.2. graphene synthesis directly on polymer
10.13.2.1. FET as carbon source patterned 30nm Ni layer as catalysator laser treatmentfor direct synthesis
10.13.3. lpcvd
10.13.3.1. large-area hbn
10.13.4. molecular beam epitaxy
10.13.4.1. graphene
10.13.4.1.1. on hBN
10.13.4.2. visualization of grain sturcture of 2d materials
11. analytics
11.1. Atomic force microscope (AFM)
11.1.1. systems
11.1.1.1. PSIA XE-100
11.1.1.1.1. tapping mode (dynamic force microscopy): - use NC-mode and NC-tip - at 5kHz adjust drive that resonance peak is in y-units 1-3 - set-point a little left of resonance peak - line set-point bit higher than half the resonance peak height best parameters: - gain: 2.2 - scan speed: 0.7 Hz
11.1.1.1.2. non contact mode (nc-mode): - use NC-mode and NC-tip - at 5kHz adjust drive that resonance peak is in y-units 1-3 - set-point a little right of resonance peak - line set-point bit higher than half the resonance peak height best parameters: - gain: 2.2 - scan speed: 0.3 Hz
11.2. scanning electron microscope (SEM)
11.3. focused ion beam (FIB)
11.4. Raman Spectroscopy
11.4.1. graphene 532nm and 633 nm laser
11.4.1.1. single layer spectrum
11.4.1.1.1. D-peak: defect peak ~1350 cm^-1
11.4.1.1.2. G-peak (4x 2D-peak): ~1580 cm^-1
11.4.1.1.3. 2D- peak: ~2700 cm^-1
11.4.1.2. multilayer spectrum
11.4.1.2.1. 5+ layer not distinguishable from graphite
11.4.1.3. suspended graphene
11.4.1.3.1. probing mechanical properties of graphene
11.4.1.3.2. ripple formation in suspended graphene
11.4.1.3.3. strain effect on suspended graphene by polarized raman
11.4.1.3.4. probing charged impurities in suspended graphene
11.4.1.3.5. intrinsic properties of exfoliated free-standing graphene
11.4.1.3.6. raman of graphene and bilayer under biaxial strain (bubbles)
11.4.1.3.7. uniaxial strain on graphene
11.4.1.3.8. elastic properties of suspended graphene
11.4.1.3.9. raman spectroscopy and kelvin force probe microscopy
11.4.1.3.10. temperature
11.4.1.4. on substrate graphene
11.4.1.4.1. nanometer-scale strain variation in graphene
11.4.1.4.2. interface coupling in twisted multilayer graphene
11.4.1.4.3. rayleigh imaging of graphene and graphene layers
11.4.1.4.4. spacially resolved raman of single- and few-layer graphene
11.4.1.4.5. graphene fingerprint
11.4.1.4.6. thickness-dependent native strain
11.4.1.4.7. surface-enhanced raman scattering of hybrid structures with ag nanoparticles ans graphene
11.4.2. MoS2 488nm laser
11.4.2.1. 382.9 cm^-1 406.0 cm^-1
11.4.2.2. on hBN
11.4.2.2.1. raman shift
11.4.2.3. mos2 on substrate
11.4.2.3.1. shift in electron irradiated monolayer
11.4.3. hBN
11.5. Keithley SCS 4200 Parameter Analyzer
11.6. Light Microscope
11.6.1. light field
11.6.2. dark field
11.7. Elipsometry
11.8. Laser confocal microscope
11.8.1. brands
11.8.1.1. Olympus LEXT OLS4100
11.9. probe station
11.9.1. Lakeshore cryo probestation
12. theory
12.1. electrical
12.1.1. resistivity: ρ = R * A / l = [ Ωm] ρ: resistivity R: resistance A = w * t: cross section area = width * thickness l: length most used: Ωmm, Ωµm
12.1.2. capacitance: C = ε_0 * ε_r * A / h = [F] C: capacitance ε_0: permitivity ε_r: relative permitivity A: area h: plate distance
12.1.2.1. dieelectric capacitance: C = ε_0 * ε_r / t_ox = [F] C: capacitance ε_0: permitivity ε_r: relative permitivity t_ox: oxide thickness
12.1.3. pull-in voltage: V_pull-in = sqrt(2/3)^3 * (k * h^3 / ε_0 * ε_r *A)) V_pull-in: pull-in voltage k: spring constant h: plate distance ε_0 permitivity ε_r: relative permitivity A: area
12.1.4. electron mobility (graphene): µ = (L / W) * g_m / C_ox * V_ds µ: mobility L: channel length W: channel width g_m provided by keithley g_m = dI_ds/dV_g at each V_gs point is calculated for each g_m point g_m = I_ds / ( V_gs - V_d) or for each g_m point g_m = (y2-y1)/(x2-x1) V_ds: source-drain voltage C_ox: oxide capacitance C_ox = ε_0 * ε_r / d_ox ε_0 permitivity ε_r: relative permitivity d_ox: oxide thickness
12.1.5. gate leakage: - parallel shift of the curve indicates a gate leakage - always check I_gate and plot it while measurement
12.2. mechanical
12.2.1. mechanical stress: σ = F / A = [N/m^2] σ: mechanical stress F: force A = w * t: cross section area = width * thickness
12.2.2. mechanical strain: ε = σ / E = s / l = [ ] ε: mechanical strain σ: mechanical stress E: young's modulus s: displacement due to mechanical strain l: length
12.2.3. force
12.2.3.1. Force (related to mechanical stress): F = A * E * ε = [N] F: force A = w * t: cross section area = width * thickness ε: mechanical strain
12.2.3.2. electrostatic force: F = 1/2 * ε_0 * ε_r * A * (V^2 / h^2) = [N] F: eletrostatic force ε_0: permitivity ε_r: relative permitivity A: area V: voltage h: plate distance
12.2.4. gauge factor: η = ΔR / ε * R η: gauge factor ΔR: change in resistance R: resistivity ε: strain: ε = (P * L / µ)^2/3 ε: strain P: pressure L: length of cavity µ: graphene shear modulus (150 N/m) --> ΔR / R = η (P * L / µ)^2/3
12.3. circuits
12.3.1. types
12.3.1.1. ring oscilator - series of at least 3 inverters (inverter: nmos+pmos) - final output of the last is inertial input in the first - final output is inverted of the inertial input - channel takes some time to charge - oscillation starts spontaneously - increase of frequency: 1. increase applied voltage 2. smaller number of inverters
12.3.1.2. inverter - 1 nmos and 1 pmos together - input voltage is inverted
12.4. units
12.4.1. electrical
12.4.1.1. E- field: N / C = V / m = kg * m / s^3 * A
12.4.1.2. Volts: V = W / A = m^2 * kg / s^3 * A
12.4.1.3. Farad: F = C / V = s^4 * A^2 / m^2 * kg
12.4.1.4. Ohm: Ω = V / A = m^2 * kg / s^3 * A^2
12.4.1.5. Coulomb: C = A * s
12.4.2. mechanical
12.4.2.1. Pascal: Pa = N / m^2 = kg / m * s^2
12.4.2.2. newton: N = m * kg / s^2
12.4.2.3. Watt: W = J / s = m^2 * kg / s^3
12.4.2.4. Joule: J = N * m = m^2 * kg / s^2 = eV * C
12.5. optical
12.5.1. wavenumber: k = 2 * π / λ = [1 / m] k: wavenumber λ: wavelength
12.5.2. wavelength: λ = 1.24 / h * ν = [µm] λ: wavelength hν: energy in eV
12.6. constants
12.6.1. mechanical
12.6.1.1. speed of light: c = 2.9981e8 m/s
12.6.1.2. atomic mass unit: u = 1.66e-27 kg
12.6.1.3. boltzmann's constant: k = 1.38e-23 J/K = 8.62e-5 eV/K
12.6.1.4. electron rest mass: m_0 = 9.11e-31 kg m_o c^2 = 5.11e5 eV
12.6.1.5. proton rest mass: m_p = 1.67e-27 kg m_p c = 9.38e8 eV
12.6.1.6. neutron rest mass: m_n = 1.67e-27 kg m_n c^2 = 9.38e8 eV
12.6.1.7. planck's constant: h = 6.63e-34 Js = 4.41e-15 eVs ћ = 1.05e-34 Js = 6.58e-16 eVs
12.6.1.8. avogadro's number: N_A = 6.02e23 molecules/mole
12.6.1.9. energy at room temperature: kT = 0.0259 eV = 4.11e-21 J = 9.83e-22 cal = 4.114 pN * nm
12.6.2. electrical
12.6.2.1. elementary charge: q = 1.602e-19 C
12.6.2.2. permittivity: ε_0 = 8.85e-12 F/m = 8.85e-15 F/cm
12.6.3. optical
12.7. midnight formula: x_1/2 = -b +- sqrt(b^2 - 4 * a * c)/2 * c
13. packaging
13.1. pressure sensor
13.1.1. polymer package
14. other material
14.1. applications
14.1.1. suspended
14.1.1.1. ru nanosheets for water splitting
14.1.2. mems/nems
14.1.2.1. pressure sensor
14.1.2.1.1. flexible pressure sensor based on pdms and elastomer film
14.1.2.2. gas sensor
15. off-topic
15.1. nanotechnology
15.1.1. nanotechnology solutions for global water challenges